General Relativity/Introduction
General relativity (GR) is a theory of gravitation that was developed by Albert Einstein between 1907 and 1915. According to general relativity, the observed gravitational attraction between masses results from the warping of space and time by those masses.
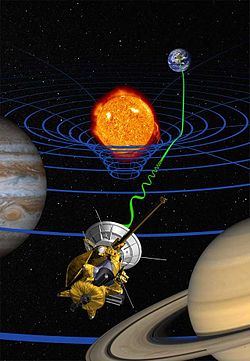
Before the advent of general relativity, Newton's law of universal gravitation had been accepted for more than two hundred years as a valid description of the gravitational force between masses. Under Newton's model, gravity was the result of an attractive force between massive objects. Although even Newton was bothered by the unknown nature of that force, the basic framework was extremely successful at describing motion.
However, experiments and observations show that Einstein's description accounts for several effects that are unexplained by Newton's law, such as minute anomalies in the orbits of Mercury and other planets. General relativity also predicts novel effects of gravity, such as gravitational waves, gravitational lensing and an effect of gravity on time known as gravitational time dilation. Many of these predictions have been confirmed by experiment, while others are the subject of ongoing research. For example, although there is indirect evidence for gravitational waves, direct evidence of their existence was also achieved by team of scientists in experiments such as the LIGO .
General relativity has developed into an essential tool in modern astrophysics. It provides the foundation for the current understanding of black holes, regions of space where gravitational attraction is so strong that not even light can escape. Their strong gravity is thought to be responsible for the intense radiation emitted by certain types of astronomical objects (such as active galactic nucleus or microquasars). General relativity is also part of the framework of the standard Big Bang model of cosmology.
Although general relativity is not the only relativistic theory of gravity, it is the simplest such theory that is consistent with the experimental data. Nevertheless, a number of open questions remain: the most fundamental is how general relativity can be reconciled with the laws of quantum physics to produce a complete and self-consistent theory of quantum gravity.
In ordinary three-dimensional space the formula for distance in Cartesian coordinates is
Now one can change the coordinate systems if one wants. If one rotates the coordinate system or stretches or shrinks it, the values for x, y, and z may change, but the distances will not. We can even conceive of more radical changes, like going into spherical coordinates where
In special relativity we learned that physics is described by another invariant in which
Again, we are free to change the coordinates of x, y, z and t to anything we want, but the underlying geometry and distances don't change.
The next step is to incorporate gravity into this picture. While the mathematical details can be complex, the basic idea is that the effects of gravity are equivalent to the effects of acceleration on an observer. From this equivalence principle, Einstein was able to show that what matter does is to change the rules for distances. The formula we showed above is strictly true only when matter is not present; when matter is present, the rules for determining distances change, and the effect of these changes is to produce the effects of gravity we all know.
This picture of gravity is powerfully simple and elegant. However, there is one problem with it; in order for it to be usable, it is necessary to learn many new mathematical concepts to understand how this picture works.
In our daily lives, we have become very familiar with the properties of three-dimensional Euclidean space because that is the world we live in. In order to do anything such as walking, moving, or catching balls, our brains have to deal with 3-space and so we have a great deal of intuitive knowledge about how this sort of geometry works. Even when we are doing mathematics in three-dimensional space, we are helped by the fact that our minds have this sort of knowledge built in. However when we discuss other types of space, our normal intuition fails us, and we are forced to follow the much more difficult path of trying to figure out what happens by describing the situation through precise mathematical statements, and this involves learning several new mathematical concepts and techniques.
To give an example of the mathematical techniques we will have to learn. Imagine you are on the surface of a flat plane. One formula for distance is
Another formula for distance in the plane in polar coordinates is
Now these two formula look quite different, but they are really two different descriptions of the same situation.
On the surface of a cylinder
we again have a formula which looks very similar to the distance in the plane expressed in polar coordinates: locally, the cylinder cannot be distinguished from the plane. Later on, we will give this a name: we call these flat surfaces.
However if we were on the surface of a sphere, then the distance for small changes in φ and θ is
Now in this situation, the difference in the distance formula is not merely one in which we are using different coordinates to talk about the same thing, the thing that we are talking about is actually different. So this brings up a lot of questions. How do we know if the differences in distance formulas are real or are just differences in coordinate systems? Can we talk about distance formulas in a way that lets us naturally distinguish between real differences rather than ones that are the result of our descriptions? How can we classify different geometries? All of this may be intuitively obvious when we are talking about three-dimensional spaces or two-dimensional objects such as spheres embedded in three dimension space. However, in order to talk about the behavior of four-dimensional space-time geometries, we need to rely on mathematical statements to get us answers.
Fortunately, mathematicians such as Riemann worked this all out in the start of the 19th century. However to understand how to deal with weird geometries, we will need to learn a few more concepts, such as the concept of a tensor.