General Astronomy/Extrasolar Planets
Exoplanets are planets that orbit other stars.
Brief History of Detecting Exoplanets
editSpeculations about the existence of exoplanets have been ongoing since the time of Isaac Newton’s General Scholium (1713). Newton hinted that other stars had planets orbiting them just as our sun. [1] The first claims of a detection of an exoplanet were centered on 70 Ophiuchi. In 1855 Capt. W. S. Jacob claimed that the orbit of the binary system exhibited an anomaly. A third body, an exoplanet, was held responsible. [2] In the 1890’s, Thomas Jefferson Jackson See supported these claims based on orbital movement as well. [3] In 1899, those claims were disproved by Forest Moulton who analyzed the triple system and demonstrated that such a system would be unstable under the orbital parameters put forth by Jackson. [4]
In the 1960’s Peter Van de Kamp claimed that Barnard's Star had an exoplanet. He based this claim on an apparent wobble in the star’s motion. [5] Kamp spent 40 years studying the Barnard star. Observations made by other telescopes were never able to replicate the date of the wobble. It is believed that the wobble was an anomaly of equipment at Sproul Observatory. There is still no conclusive evidences for or against a planet existing around Barnard’s Star. [6]
The first detection to be later confirmed of an exoplanet was made in 1988 by Bruce Campbell, G.A.H. Walker and S. Yang. The star Gamma Cephei was tentatively proposed to have an exoplanet based on radial velocity observations. It was given a tentative status because the observations were made at the very limit of the capability of the instruments at the time. [7] The claim was mistakenly retracted, for in 2002 Artie Hatzes and his collaborators were able to confirm the existence of the exoplanet. [8]
In 1992 Wolszczan and Frail proved there were two planets of 3.9 and 4.3 Earth masses orbiting the pulsar PSR B1257+12 at .46 and .36 AU respectively. [9] A third planet was discovered later of .025 Earth masses orbiting at .19 AU. This discovery is considered to be the first definitive detection of an exoplanet, because the Cambell, Walker, and Yang discovery was not confirmed until 2003. This was a rather strange discovery because it was revolving around a pulsar rather than a main sequence star like our sun. It is believed that the planets are the remains of a solar system after the star died. [10]
The first discovery of an exoplanet orbiting a main sequence star, 51 Pegasi, was announced October 6, 1995 by Michel Mayor and Didier Queloz. [11] When the distance and mass of the planet were determined they were found to be incompatible with theories of planetary formation at the time. [12] They used the radial velocity method to detect the planet. This ushered in the modern era of exoplanet study. After their discovery numerous exoplanets have been detected as a result of improved telescopes with higher resolutions and more powerful computers. [10]

As of August 19, 2012, 777 exoplanets have been detected. [13] The rate of discovery has steadily increased due to improved technology and a greater interest in exoplanets, with 61 planets detected in 2007. Using the Spitzer Space Telescope, studies of the evolution of gas and dust around main sequence stars indicate that at least 20 percent, and possibly as many as 60 percent, of main sequence stars are candidates for forming rocky planets. The actual percentage is likely between these two extremes. [14] [15]
Most exoplanets detected have been massive Jupiter-like planets. Most likely this is due to the ease of detecting such in comparison to smaller terrestrial planets; this is known as a selection effect. Planets, labeled Super-Earths, have also been detected. They are about 5 to 10 Earth masses. One Earth mass would be the mass of the earth. [16]
Detection Methods
editThere are six methods currently used to detect exoplanets: Astrometry, Radial Velocity, Pulsar timing, Transit method, Gravitational micro-lensing, Direct imaging. Three methods detect a dynamical perturbation of the star, or wobble of the star due to the planet’s gravitational force. Since the planet and the star revolve around a common center of mass the star will appear to wobble with the same period as the planet’s orbital period. From our point of view the wobble will be from side to side, towards and away, or some combination of the two.

The degree of a star’s wobble is determined by the mass of the star and the planet, and by the distance between them. The more massive a planet is in comparison to that of its star, the greater the wobble. The closer the planet’s orbit to the star the greater the degree of the wobble observed of the star. Any method that detects an exoplanet this way can give information about the planet’s mass, though a combination of methods is needed to find the true mass of a planet.

The three ways we detect this wobble are by radial velocity, astrometry, and pulsar timing. Radial velocity measurements detect the blue and red shifts of a star as it moves toward and away from Earth, respectively. As the star moves toward Earth, the light shifts to blue. As the star moves away from Earth the light shifts to red. The radial velocity can be deduced by studying the red and blue shifts in the spectroscopy of the star’s emission. Most exoplanets have been discovered by the radial velocity method. [17]
The velocity of the star around the barycenter is much smaller than the planet’s velocity because the planet’s orbital distance from the center of mass is greater than the star’s. Current spectrometers can detect a velocity variation down to 1 m/s. [18] The larger the velocity differences in the star, the easier it is to detect. Thus the radial velocity method can more easily detect exoplanets that have a larger mass and are closer to their stars. [17]
The Astrometry Method is the oldest search method though it wasn’t the method used to discover the first exoplanet. The astrometry method looks for angular change in the position of a star. The more a star moves, the easier it is to detect. Since this method relies on the orbit around a center of mass it is dependent on the mass ratio of the planet and star. [17]
However, unlike the radial velocity, the angular change in position increases with greater distance between the star and the exoplanet. The further the planet is from the star, the further they are from their mutual center of mass. The greater a star’s distance from the center of mass, the greater the angle change between the star’s opposite ends of its orbit. [17]

In the Pulsar Timing Method, a pulsar emits radio waves in extremely regular time intervals as it rotates. Any anomalies in the time between radio waves are an indication that the pulsar is orbiting a center of mass. This method is very sensitive. Planets that are one-tenth Earth masses can be detected this way.[17]

The Transit Method detects changes in the brightness of star as a planet moves across the star's face. The dimmer the star becomes, the larger the planet, thus the volume of a planet can be determined by this method. This method requires that the planet’s orbit passes directly between the star and earth, which is a rare alignment.[17] The Gravitational Lensing Method measures an amplification of a star’s brightness. As light from a background star passes the planetary system, the light is bent towards a planet due to its

gravity. The light is focused into a smaller area thus increasing its brightness. This method is capable of detecting low mass planets around dim stars at a great distance.[19]
The final method currently used to detect planets is to directly observe them. Usually a parent star's shine obscures a planet's emission. However, in certain cases the reflected light of a planet can be seen. Such a method works best when the planet is more massive than Jupiter, less than a tenth the age of our solar system, more distant from its parent star than Jupiter and orbits a star less luminous than our sun. It is also possible to detect a planet’s light if we restrict ourselves to infrared radiation, which is the dominant emission wavelength of a planet. This is very difficult and few planets have been detected this way.[10]
Extrasolar Jovians
editMany gas giants have been discovered orbiting distant stars, many of larger than Jupiter. That is because big planets are massive enough to gravitationally affect the movement of their star sufficiently that the perturbation is detectable. Therefore the biggest extrasolar planets are the easiest and earliest to be found.
The First Discovery
editThe first extrasolar planet discovered was Gamma Cephei Ab, a planet orbiting Gamma Cephei A with an orbital period of around 900 days at a distance of around 1.7 - 2.5 AU from its parent. Its existence was suspected as early as in 1989, and evidence of it strengthened considerably in 2002 from observations at the McDonald Observatory. It is a Jovian-sized planet with a mass estimated to be above 1.59 times that of Jupiter's. The star it orbits is an orange sub-giant star part of the Gamma Cephei binary star system, with its companion Gamma Cephei B a red dwarf.
Spectra of Hot Jupiters
edit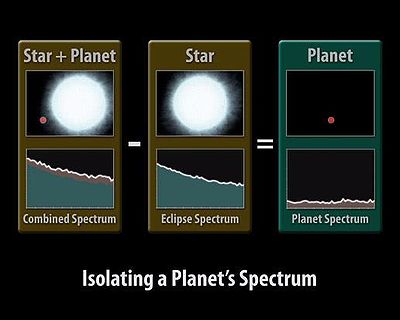
Astrophysicists have recently observed spectra from hot Jupiter sized planets in planetary systems many light years away.[20] Since these planets are so distant, it’s not as simple as just looking at the planet and collecting data about the direct light from the planet.[21] The planets are too distant for such techniques and they are too close to their parent stars (sometimes closer than Mercury is to our sun).[21] Instead astrophysicists collect data from the star and planet during different parts of the planets’ orbit and separate the light from just the planet.
First the spectrum from the planet and star is collected. This is done when, as seen from earth, both planet and star are at their greatest separation. Then the spectrum of just the star is obtained. This is done when the planet is either directly in front of the parent star (primary eclipse) or directly behind its parent star (secondary eclipse). By subtracting the light of the star from the light of the star plus planet, the spectrum of the planet can be found.[21]

Spectroscopic Techniques: Primary and Secondary Eclipses
editThe brightness of the parent stars of these planets is too high in comparison to the planets themselves in the optical range. Because of this, infrared light is detected to better differentiate the planet from the star.[21] The planet itself reflects very little optical light because the cloud layers prevent much of it from escaping. The cloud layers emit much of the absorbed energy from its star as infrared light however, and so most it can be detected by the spectrometers of our telescopes (Spitzer and Hubble). Because the planet emits so much more radiation in the infrared range as compared to the reflected optical part of the spectrum, the differences in the two spectra are more exaggerated and the infrared part of the spectra is easier to use.
A secondary eclipse occurs when the hot Jupiter being observed passes behind its parent star, as viewed from Earth. A primary eclipse, also known as a transit, happens when the planet passes in front of the star as viewed from Earth. These eclipses are very rare because the planet, star and earth have to line up so that the planet completely eclipses.[21] Only 54 of the 333 confirmed planets are transiting planets when viewed from Earth.[22]
Spectroscopic results
editSpectra are used to identify molecules in the planetary atmospheres, and to determine relative abundances. If you know at what wavelengths an atom or molecule will emit or absorb light, you can examine the spectrum of light from a planet and determine if that molecule is present. By looking at the full spectrum, scientists can decide which atoms or molecules specifically make up the planet and noting how much of that wavelength is emitted, the relative abundance of that atom or molecule can also be determined.

Many attempts are focusing on identifying water vapor on other planets.[21] The two planets, HD 209458b and HD 189733b are the first two planets observed.[20] Originally, they both seemed to be void of water vapor.[21] It is believed that the observational techniques weren’t sensitive enough to detect water vapor. By using new techniques, the evidence for these planets containing water vapor is growing.[23] The first tests were done using a secondary eclipse of the star.[21] Because of the atmospheric composition of the planets, this technique could not see through the clouds on the planets. The heat of the planet due to its closeness to the star also plays a part in the differences in results. Because the planet is so close to the star, the side closest to the star will have less water than the side away from the star. During a primary eclipse, the cooler and therefore wetter side will be facing towards earth. During a secondary eclipse, the dryer hotter side will be facing towards earth. By using a primary eclipse,[24] a team of scientists in France has found peaks in the light curve at 3.6 microns, 5.8 microns and 8 microns in such a pattern that they believe only water vapor could produce.[20]
Example Spectra
edit


References
edit- ↑ Isaac Newton, The Mathematical Principles of Natural Philosophy, trans. Andrew Motte (London, 1729), pp. 387-93.
- ↑ Jacob, W. S. "On certain Anomalies presented by the Binary Star 70 Ophiuchi." Monthly Notices of the Royal Astronomical Society 15 (1855): 288-89. http://adsabs.harvard.edu/abs/1855MNRAS..15..228J
- ↑ Thomas Jefferson Jackson (1896). "Researches on the Orbit of F.70 Ophiuchi, and on a Periodic Perturbation in the Motion of the System Arising from the Action of an Unseen Body". The Astronomical Journal 16: 17. http://adsabs.harvard.edu/cgi-bin/bib_query?1896AJ.....16...17S
- ↑ Sherrill, Thomas J. (1999). "A Career of controversy: the anomaly OF T. J. J. See" (PDF). Journal for the history of astronomy 30, http://www.shpltd.co.uk/jha.pdf. Retrieved on 27 August 2007.
- ↑ van de Kamp, Peter (August 1969). "Alternate dynamical analysis of Barnard's star". The Astronomical Journal 74: 757–759. doi:10.1086/110852, http://adsabs.harvard.edu/abs/1969AJ.....74..757V. Retrieved on 27 August 2007
- ↑ Bell, George H. "The Search for the Extrasolar Planets: A Brief History of the Search, the Findings and the Future Implications." 5 Apr. 2001. Arizona State University. Nov. 2008 <http://www.public.asu.edu/~sciref/exoplnt.htm#preface>.
- ↑ Campbell, B.; Walker, G. A. H.; Yang, S. (1988). "A search for substellar companions to solar-type stars". Astrophysical Journal, Part 1 331: 902 – 921. doi:10.1086/166608, http://adsbit.harvard.edu/cgi-bin/nph-iarticle_query?bibcode=1988ApJ...331..902C
- ↑ Hatzes et al. (2003). "A Planetary Companion to Gamma Cephei A". The Astrophysical Journal 599 (2): 1383 – 1394. doi:10.1086/379281, http://www.journals.uchicago.edu/doi/full/10.1086/379281
- ↑ Wolszczan, A.; Frail, D. A. (1992). "A planetary system around the millisecond pulsar PSR1257+12". Nature 355: 145 – 147. doi:10.1038/355145a0, http://www.nature.com/nature/journal/v355/n6356/abs/355145a0.html
- ↑ a b c Dr Guyon, Olivier "A brief history of the search for extrasolar planets." 11 June 2006. Scitizen. Nov.2008 http://www.scitizen.com/screens/blogPage/viewBlog/sw_viewBlog.php?idTheme=7&idContribution=18
- ↑ Mayor, Michel; Queloz, Didier (1995). "A Jupiter-mass companion to a solar-type star". Nature 378: 355 – 359. doi:10.1038/378355a0, http://www.nature.com/nature/journal/v378/n6555/abs/378355a0.html
- ↑ Mayor et al. (1995). "A Jupiter-mass companion to a solar-type star". Nature 378: 355–359. doi:10.1038/378355a0
- ↑ Schneider, Jean (19 September 2012). "Interactive Extra-solar Planets Catalog" . The Extrasolar Planets Encyclopedia. Retrieved 2012-08-19.
- ↑ "Many, perhaps most, nearby sun-like stars may form rocky planets." Innovations-report. 19 Feb. 2002. <http://www.innovations-report.de/html/berichte/physik_astronomie/bericht-103754.html
- ↑ Hillenbrand, Lynne A., John M. Carpenter, Kim Jinyoung Serena, Michael R. Meyer, Dana E. Backman, Moro-Martín, Amaya, David J. Hollenbach, Ilaria Pascucci, Dean C. Hines, and Jeroen Bouwman. "The Complete Census of 70 μm-bright Debris Disks within the Formation and Evolution of Planetary Systems Spitzer Legacy Survey of Sun-like Stars." The Astrophysical Journal 677 (2008): 630-56. http://adsabs.harvard.edu/abs/2008ApJ...677..630H
- ↑ Valencia, Diana, Dimitar D. Sasselov, and Richard J. O'Connell. "Radius and Structure Models of the First Super-Earth Planet." The Astrophysical Journal 656 (2007): 545-51. http://adsabs.harvard.edu/abs/2007ApJ...656..545V
- ↑ a b c d e f Henry, Gregory, Eric Ford, Chris McCarthy, Kevin Apps, Greg Laughlin, and Bernie Walp. California & Carnegie Planet Search. Anglo-Australian. <http://exoplanets.org/><http://exoplanets.org/othermethframe.html>
- ↑ Wright, Jason T. "Detecting Exoplanets with Precise Radial Velocities."
- ↑ Sackett, Dr. Penny D. "Microlensing exoplanets." 5 Aug. 2008. Http://www.scholarpedia.org/article/Microlensing_exoplanets.com
- ↑ a b c Sing, D. 2008, Astrophysical Journal 686:658–666, 2008 October 10
- ↑ a b c d e f g h Thompson, T. 2007, NASA Press Release http://www.nasa.gov/mission_pages/spitzer/news/spitzer-20070221-full.html
- ↑ Schneider, J. 2008, Extrasolar Planets Encyclopedia, http://exoplanets.eu
- ↑ Barman, T. 2007, ApJ, 661, L191
- ↑ Tinetti, G. et al. 2007, Nature, 448, 7150, 169